By Salam Murtada, Daniel Reinartz, and Steve Kloiber
OVERVIEW
Cover crops provide many benefits that include improving soil health, providing storage to reduce runoff, preventing soil erosion, and protecting water quality. They benefit both the environment and farm operators by optimizing the use of fertilizers, allowing runoff treatment through the unsaturated soil, and preventing the loss of nutrients to the rivers.
Cover crops are typically applied between harvest and growing seasons. They can also be interseeded with cultivated crops during the growing season. However, they have the greatest potential benefit in early spring, before planting, when the ground is otherwise fallow and soil is vulnerable to intense rainfall events.
This study compares the effects of cover crops between growing and non-growing seasons and investigates the processes that drive them, using continuous and synthetic events simulations. These processes include infiltration, surface runoff, storage capacity, and soil moisture. This paper quantifies the benefits of cover crop application in the Shakopee watershed, Minnesota through hydrological modeling using the Gridded Surface Subsurface Hydrological Analysis (GSSHA) model.
Haruna et al., 2020, summarized studies published in the last 20 years addressing the benefits of cover crop on soil physical properties. According to these studies, cover crops significantly reduced soil density and increased soil organic content leading to reductions in soil loss and surface runoff, and increases in water holding capacity, infiltration and potential carbon sequestration. In another study, Basche and DeLong, 2017, presented the benefits of cover crops and other continuous living crops in combating rainfall infiltration variability, after statistically determining their effects on increasing porosity (8.0 + 2.2%) and soil water retention at field capacity (9.3 + 2.7).
The Gridded Surface Subsurface Hydrological Analysis (GSSHA) model is a physically-based, distributive model that simulates the interactions between the complex hydrological processes taking place on the surface and subsurface media at fine temporal and spatial scales. It uses finite difference in space to the second order, and forward difference in time to the first order. The model interfaces with the Watershed Modeling System (WMS) graphical user interface. GSSHA was developed by Dr. Charles Downer of the Environmental Research and Development Center (ERDC), United States Army Corps of Engineers (USACE) and is currently supported by Aquaveo, Inc.
The Shakopee watershed (323 mi2) extends across three counties: Kandiyohi, Chippewa, and Swift counties in central Minnesota (Figure 1). It is located in predominantly agricultural areas, where corn and soybean crops comprise approximately 68% of the total watershed. The soils are primarily fine-textured and poorly drained.
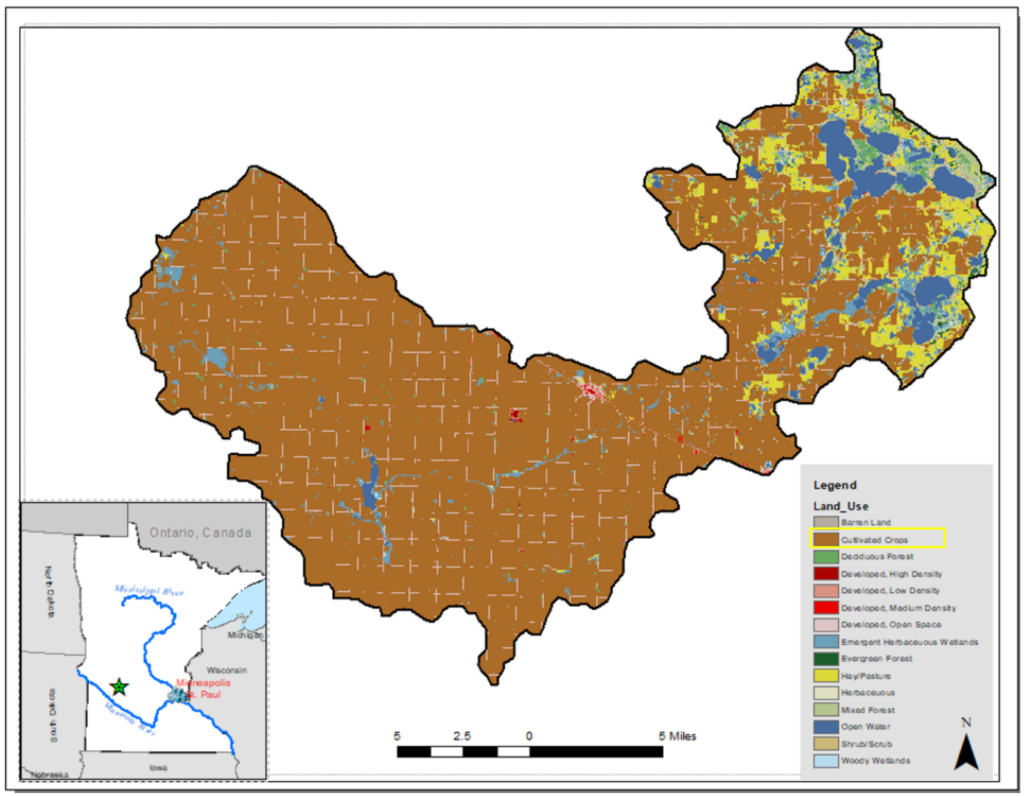
METHODS
In this study, the effects of cover crops on the subsurface and surface processes were simulated to evaluate changes to surface water runoff, infiltration, and evapotranspiration. After calibrating and validating the model, cover crops were introduced as a hypothetical scenario in the form of small grains applied over all agricultural areas during growing and non-growing seasons. Examples of small grains are winter-hardy cultivars of rye, wheat, and triticale that can survive cold weather. Input climate variables were held constant in the model.
Large grid cells (9.88-acre) were used to accommodate the computation time to simulate the large, 323 square-mile watershed. The model consists of three layers to represent the upper tillage layer and lower soil layers as reported by the Soil Web. Key model assumptions and parameters are summarized in Table 1.
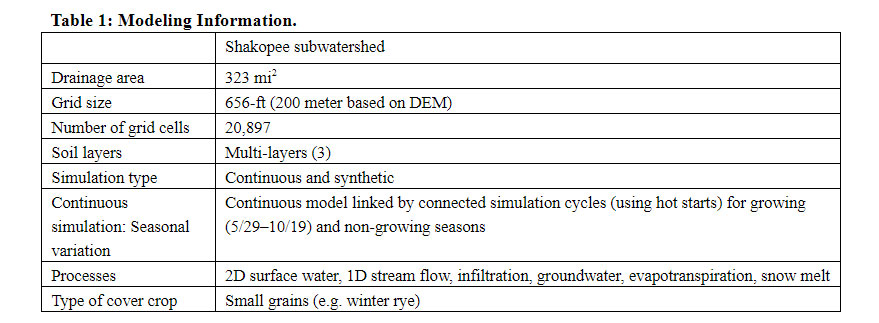
The simulation was broken down into two connected and continuous cycles, non-growing and growing, where different processes controlled the hydrology. This enabled us to compare the effects of cover crops between the two cycles in order to highlight their benefits more accurately (Figure 2).
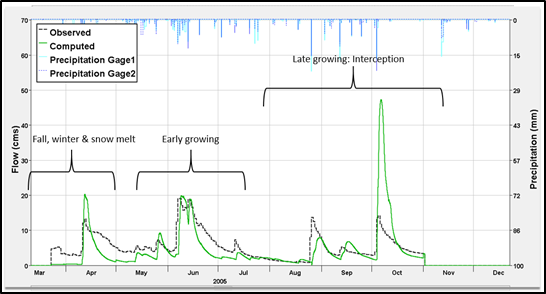
The model simulated the effects of cover crops as follows:
Hydraulic Conductivity
Hydraulic conductivity is an important factor to characterize the effects of cover crops. Based on cover crop ability to decrease soil compaction and increase the organic content of the soil within its root zone, empirical relationships from the Water Erosion Prediction Project (WEPP) method (USDA-WEPP, 1995) were used to determine the saturated hydraulic conductivity. The WEPP method combines the effects of soil properties based on the Hydrologic Soil Group (HSG) and soil texture, as well as land use features based on a specific agricultural practice (e.g. conservation tillage) or the curve number (CN). The model used the hydraulic conductivity to simulate infiltration using the Green & Ampt method. Soil textures were obtained from the Soil Survey Geographic Database (SSURGO) to compute the other parameters using Saxton & Rawls equations (2006) and Brooks & Corey equations.
Roughness
Roughness characterizes the ability of cover crops to slow water flow and create micro-storage on the surface. By increasing residency time due to roughness, cover crops increased storage and allowed more time for water to infiltrate. The model included roughness to simulate surface water flow using the Diffusive Wave equation.
Evapotranspiration (ET)
The model used the Penman-Monteith equation, where the canopy stomatal resistance and vegetation heights were adjusted to account for the effects of small grains. In addition, the evapotranspiration was adjusted seasonally in the model through a multiplier called the Canopy Resistance Amplification Factor that increased the stomatal resistance during the winter months and decreased it to 1.0 during the growing season.
Interception and Retention
These parameters were used in the late growing season to account for canopy effects in intercepting rain and retaining it on the surface.
RESULTS AND DISCUSSION
Runoff volume reduction
According to this study, the application of cover crops reduced discharge volume at the watershed outlet by an average of 11% and 41% for growing and non-growing seasons, respectively (Figure 3). Cover crop applications achieved maximum benefits during the non-growing season after they were compared with fallow ground conditions. This was attributed primarily to increases in the saturated hydraulic conductivity and roughness by 2 and 3 times, respectively. In the growing season, however, the cover crops were competing with the cultivated crops, showing improvements based on hydraulic conductivity, but not necessarily roughness. As a result, surface runoff reduction due to cover crop application was higher for the non-growing season than the growing season by a factor of 3.6 (Figure 3). Furthermore, the rate of infiltration increase due to cover crop application was higher for the non-growing season by a factor of 4.7 (Figure 3).
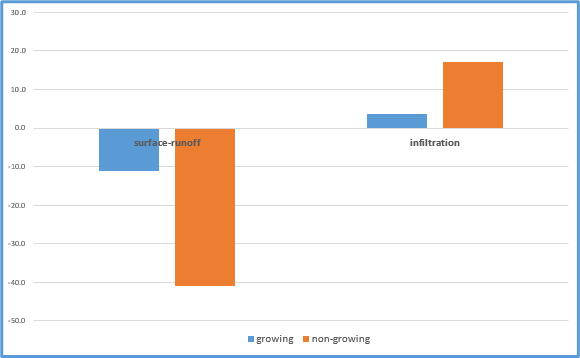
Effects of cover crops on storage
According to the model results, extensive cover crop application removed an average of approximately 17,500 acre-feet of runoff volume computed at the watershed outlet (Figure 4) (equal to approximately 1-inch of runoff over the watershed) during the non-growing season. Most of the net volume removed infiltrated through the soil during the fall and early spring when the ground was not frozen. Water storage was spatially distributed across the landscape, yielding significant cumulative benefits at the watershed outlet (Figure 5).
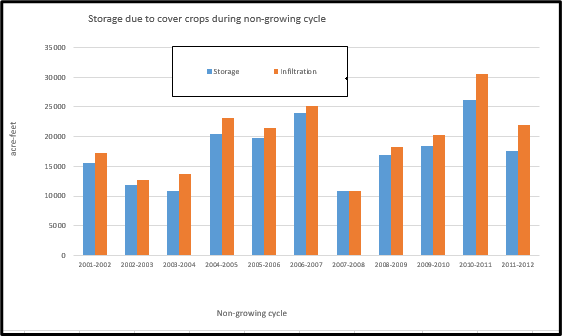
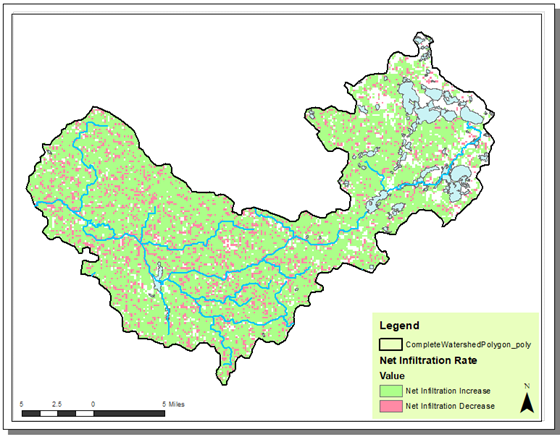
Peak flow and discharge volume sensitivity to model parameters
The simulation for the non-growing period was broken down further into separate simulations to investigate the influence of different model parameters on both peak flows and the discharge volume. Model parameters for hydraulic conductivity and roughness were analyzed independently using synthetic rainfall events. The results showed that peak flow was reduced by 30% from the combined effects of roughness and hydraulic conductivity. Discharge volume was reduced by 24%, but the relative importance and the interaction between hydraulic conductivity and roughness were different. Hydraulic conductivity and roughness were both important in reducing the volume of discharge when considered separately. However, their effects did not combine as they did for peak flow reduction. For peak flow reduction, the rate of infiltration alone is overwhelmed by the rate of precipitation. However, an increase in roughness would help slow the flow and give it more residence time for infiltration to occur. For volume reduction, both parameters are as important independently and in combination, because they affect storage on the surface as well as the subsurface in the form of recharge into the groundwater or plant uptake. In the end, the total volume at the watershed outlet will also include infiltrated flows that will ultimately exfiltrate into the stream network as groundwater baseflow.

Conclusion
Using the GSSHA model, this study quantified and characterized the water storage benefits of cover crop application for reducing both peak flow and volume discharge. It highlights the importance of cover crops as a potential tool for flood mitigation and watershed management.
The results of the analysis presented here show that the hydrologic benefits of cover crops are particularly important for the non-growing season. Cover crop applications in the non-growing season produced significant reductions in discharge volume of up to 41% when compared to fallow ground conditions. The GSSHA simulation also demonstrated volume reductions up to 11% in discharge volume for the growing season.
Both saturated hydraulic conductivity and surface roughness were the main drivers in controlling the peak flow and volume of discharge reductions, according to the simulation. Their combined effects amplified peak flow reductions.
Author Details
Salam Murtada is a civil and environmental engineer working as floodplain hydrologist for the Minnesota Department of Natural Resources. His job includes developing and reviewing hydrological and hydraulic models for watershed studies, FEMA and flood related projects, and geomorphic evaluation of culvert designs and stream restoration projects. He graduated from West Virginia University with a Master of Science degree in Civil and Environmental Engineering, and from the University of Texas at Austin in Bachelor of Science degrees in Civil Engineering and Petroleum Engineering.
Daniel Reinartz has worked for the Lake Ecology Unit of the Minnesota Department of Natural Resources for the past 9 years. He retired from the U.S. Army Corps of Engineers as a Hydrologic Engineer after 35 years. He has a total of 49 years in civil engineering with a BCE Degree from the University of Minnesota.
Steve Kloiber supervises the Lake Ecology Unit of the Minnesota Department of Natural Resources. He has over 30 years of experience in water resource science and environmental analysis. He received his masters and PhD from the University of Minnesota in environmental engineering with a minor in water resources science.
References
- Agricultural Research Science. (2009) Soil Water Characteristics (SWC). USDA.
- Agricultural Research Service. (1995). Water Erosion Prediction Project (WEPP). Chapter 7, Soil Component.
- Basche, A. D., DeLonge, M. (2017). The Impact of Continuous Living Cover on Soil Hydrologic Properties: A Meta-Analysis. Agronomy & Horticulture, Faculty Publications.
- Downer, C. W., Ogden, F. L., Byrd, A. R. (2008). GSSHAWIKI User’s Manual, Gridded Surface Subsurface Analysis Version 7.13 for WMA 10.1. ERDC Technical Report. Engineer Research and Development Center, Vicksburg, Mississippi.
- Haruna, S., Anderson, S. H., Udawatta, R. P., Gantzer, C. J., Phillips, N. C., Cui, S., Gao, Y. (2020). Improving soil physical properties through the use of cover crops: A review. Agrosystems, Geosciences & Environment.
- Singer, J., Kaspar, T., Pederson, P. (2005). Small Grains Cover Crops for Corn and Soybean. Iowa State University Extension and Outreach.
- Minnesota Geospatial Commons. (2013). https://gisdata.mn.gov/dataset/quick-layers
- US Army Corps of Engineers. HEC-DSSVue Version 1.2. (2006).
- University of Minnesota Extension. (2021). Cover Crops. https://extension.umn.edu/soil-and-water/cover-crops